Induced Pluripotent Stem Cells (iPSC’s) are a type of stem cell that can be used as a replacement for embryonic stem cells in the research and treatment of human disease. As a Biomedical Science student who has an interest in pharmacology and drug therapies, I believe iPSC’s will lessen our reliance on conventional drugs by targeting diseases at their root cause: cellular dysfunction. The ability to replace damaged cells with new ones provides a novel mechanism for treating diseases, but is it the one size fits all solution to all of humanities ailments?
Introduction
iPSC’s are derived from normal human cells, such as fibroblasts, and are cultured with pluripotency inducing factors such as Oct4/Klf1/Sox2, which are delivered to the cells within a viral vector. This gives the cells the properties of potency and self-renewal that are indicative of pluripotent stem cells. They can then be differentiated into tissues that can be used for drug screening in the lab or inserted into the patient in order to replace missing or damaged cells/tissues. These cells are similar in properties to embryonic stem cells (ESC’s), which are the staple type of stem cell used in regenerative medicine. However due to ethical concerns over the use of ESCs from failed IVF cycles, iPSC’s offer a more ethically sound alternative to be used in regenerative medicine.
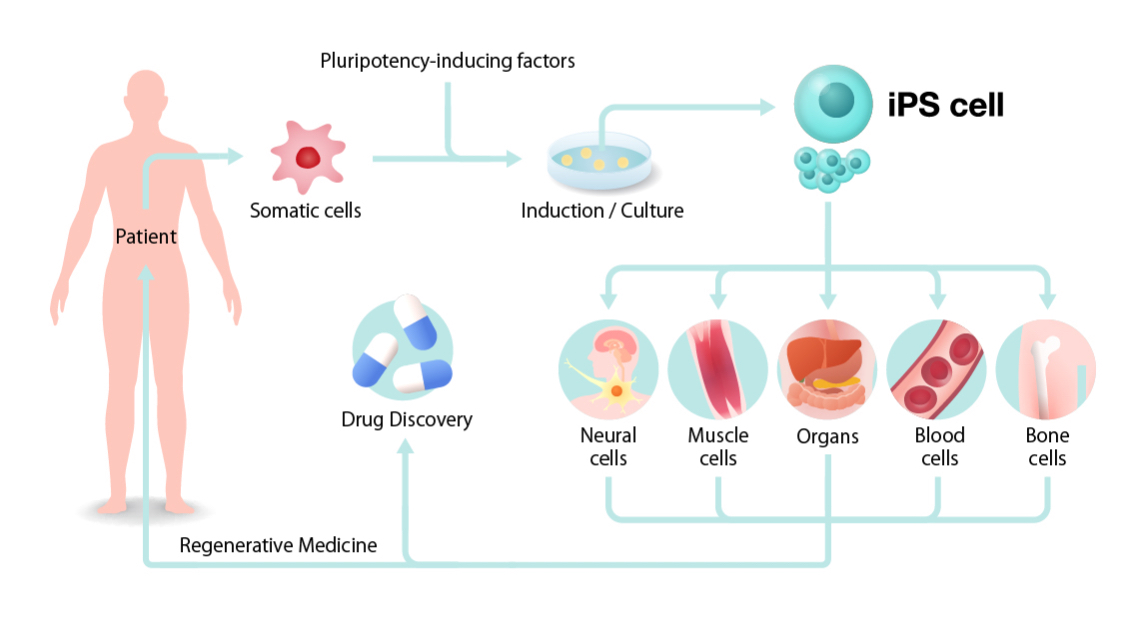
A Simplified diagram of the conversion of somatic cells into iPSC’s
The potential
iPSC’s have the potential to cure a wide range of diseases, from replacing β-cells in the pancreas in Type 1 Diabetes to the replacement of dopaminergic neurones in the brain in Parkinson’s Disease. Diabetes is important to myself as I have many family members who suffer from Type 1 and Type 2 Diabetes, and from interviews with them I found that they struggle with the insulin injection as well as the constant measuring of blood glucose level via a finger prick test. So as a future medical researcher, iPSC’s excite me, as they open an avenue to where diabetes can be cured by replacement of lost β-cells, leading to an eradication of the hampered quality of life people with diabetes must undergo.
Explores the role of stem cells in diabetes treatment.
Explores the role of stem cells in diabetes treatment.
The Issues: Ethical
iPSC’s and their side effects do not line up with Aristotle’s view of ethics as a virtue; as they would not be classified as a high-quality treatment due to their tendency to form tumours, therefore its poor ability to carry out its regenerative function would prove them to not be an effective treatment option. As well as this, in my opinion the use of these cells in a widespread manner may lead to opening pandoras box, where people will look to improve the function of their healthy tissue instead of treating diseased tissue. For example, certain factors may be able to be added to iPSC’s in order to produce muscle tissue with an abnormally high amount of Type IIx muscle fibres, and once transplanted giving them a predisposition to being a successful power athlete such as a sprinter. This in turn will unlock the door to ‘perfect’ humans, a dystopian world with a socioeconomic divide between people who can afford to improve their bodies and those who cannot.
Conclusion
To conclude, iPSC’s are very promising and have a lot of potential for use in regenerative medicine. However, the technology still needs refining and legislations need to be put in place to ensure the technology is not applied outside of disease therapy.
Very well written, with an excellent format and images. You’ve included interesting statistics and related it to personal ideas which…
This is a very well written blog, the format is as if you are talking directly to me. The ideas…
Love the Batman GIF :)
This is an excellent, well written blog. The narrative is engaging and easy to follow. It could be improved by…
This is a well-communicated blog. The it is written well with good use of multimedia. It could be improved with…