Imagine a world where baldness and hair loss is no longer a source of insecurity; a world where those suffering from these can regain their confidence and self-esteem.
Hair bioprinting isn’t just about aesthetics. It holds promise for treating various hair and scalp-related conditions, such as alopecia, a condition that affects millions worldwide. By harnessing the regenerative potential of cells, this technology may offer hope to those who have been struggling with hair loss for years.
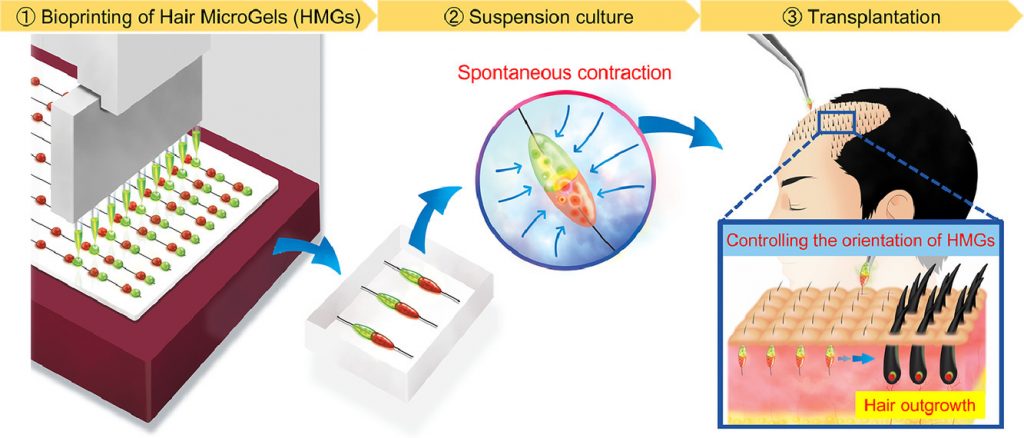
In one specific study, the replication of in-vivo tissue configurations and microenvironments like hair follicle germs has been studied to prepare tissue grafts for hair regenerative medicine. This study suggests an approach for the scalable and automated preparation of highly hair-inductive tissue grafts using a bioprinter.
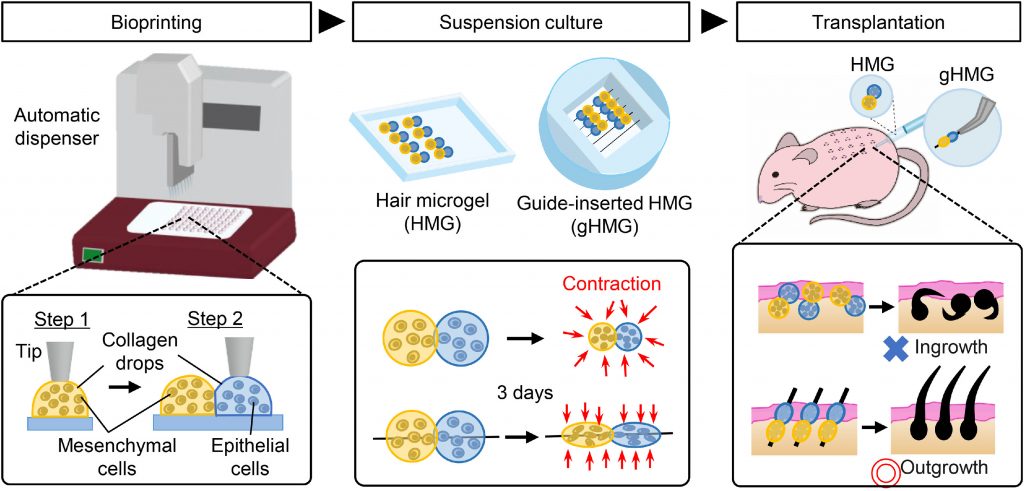
Hair follicle morphogenesis initiates with the formation of a primordium composed of mesenchymal and epithelial cells, instigating tissue development. Distinguished from other organs, the hair follicle generates the hair follicle germ (HFG) at regular intervals postnatally, perpetually renewing throughout life. In the pursuit of advancing hair regenerative medicine, diverse methodologies have been devised for engineering HFG-like grafts, with one of the most sophisticated methods being the use of bioengineered HFGs. This method entails the compartmentalization of mesenchymal and epithelial cell aggregates to elicit interactions, thereby fostering efficient hair follicle regeneration and establishment of connections with host arrector pili, nerve fibers, and recurring hair cycles upon transplantation. However, this approach may be challenging to scale up to the human setting due to laborious manual preparation steps, especially considering the need for thousands of tissue grafts for a single patient. So, there exists a need for a scalable, highly hair-inductive, and preferably automated approach for HFG preparation.
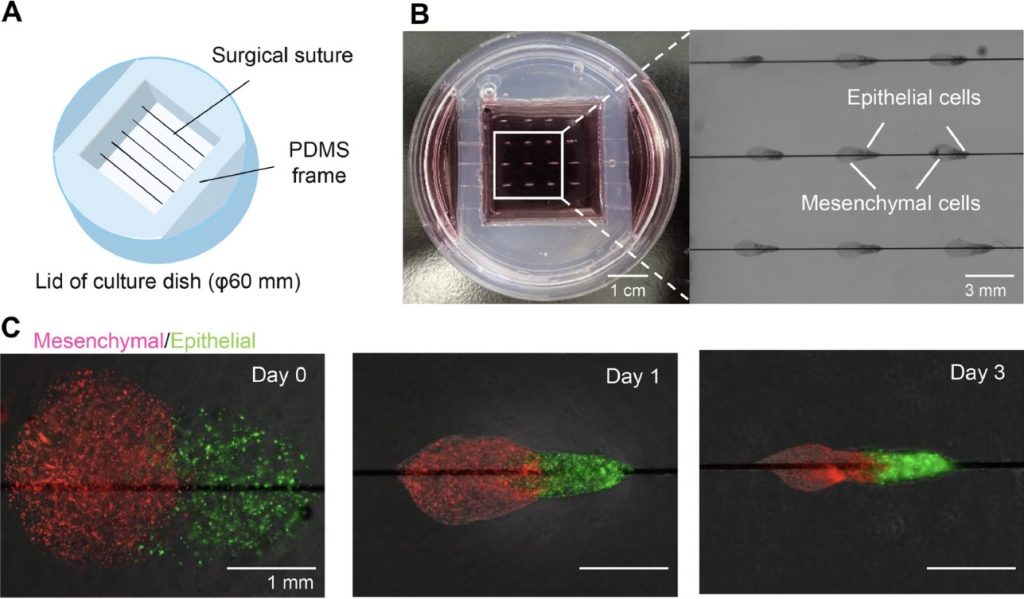
Exploration into scalable methodologies for preparing mesenchymal and epithelial aggregates has predominantly centered around the concept of cell self-organization. Among these approaches, one notable method entails the seeding of a blend of these cellular components onto a flat substrate. This process gives rise to the formation of spherical aggregates characterized by a mesenchymal core enveloped by an epithelial shell. However, while promising, this technique has encountered a notable challenge: the wide distribution of spheroid sizes. This variability in size could potentially lead to unpredictable outcomes in terms of hair-inducing functionalities. It’s been observed that the size of these spheroids correlates with the number of hairs generated, hinting at the intricacies of the relationship between structure and function in tissue engineering endeavors.
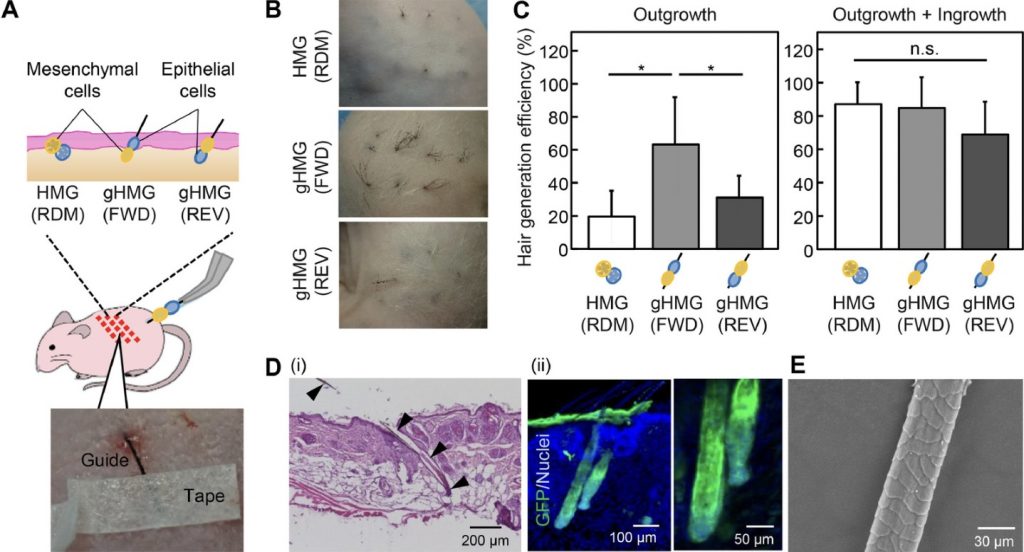
Pairs of collagen droplets housing mouse embryonic mesenchymal and epithelial cells were meticulously positioned adjacent to each other and sequentially underwent gelation. In the subsequent suspension culture, the microgel beads spontaneously contracted, thereby enriching the density of collagen and cells after three days of culture. The contracted microgel beads were termed HMGs or hair microgels. To evaluate their hair-inducing potential, they were transplanted into the dorsal skin of nude mice. gHMGs were fabricated in the same manner, but by placing pairs of collagen droplets on aligned surgical suture guides. The effects of the guides were examined after transplantation into the nude mice.
Fast forward to 2017, where the beauty giant L’Oréal made headlines by partnering with the biotech firm Poietis, renowned for its expertise in crafting 3D models of human tissues, to embark on the groundbreaking endeavor of bioprinting hair follicles. Hair bioprinting is a prime example of interdisciplinary collaboration. It brings together experts from fields like biology, engineering, and medicine, all working together to unlock the potential of this cutting-edge technology.
While the prospect of bioprinting hair follicles holds great promise, further refinement of the process is required and extensive research is needed to ensure its safety and long-term effectiveness. On one hand, the idea of being able to customize hair growth feels like a step towards empowerment, a chance to redefine our appearance according to our own desires. But on the other hand, it raises questions regarding the accessibility and affordability of this technology to a broader demographic that must be addressed, underscoring the importance of making cutting-edge advancements in regenerative medicine inclusive and sustainable. Who will have access to this technology? Will it be reserved for the privileged few, widening the gap between those with the finance and those who don’t? Or will it be made accessible to all, ensuring that advancements in science benefit everyone? It’s not just about innovation; it’s about equity and justice. As we navigate the uncharted territory of bioprinting, we must ensure that it’s not just a luxury for the elite, but a tool for empowerment and inclusivity. Only then can we truly harness its transformative potential for the betterment of all.
Very well written, with an excellent format and images. You’ve included interesting statistics and related it to personal ideas which…
This is a very well written blog, the format is as if you are talking directly to me. The ideas…
Love the Batman GIF :)
This is an excellent, well written blog. The narrative is engaging and easy to follow. It could be improved by…
This is a well-communicated blog. The it is written well with good use of multimedia. It could be improved with…