Approximately 45% of the mass of the human adult body is muscle tissue.
Serge Ostrovidov, PhD et al.
Muscles are critical in locomotion, prehension, mastication, ocular movement, and other dynamic activities such as body metabolic regulation. Myopathy, traumatic injury, aggressive malignant tumour extraction, and muscular denervation are the most prevalent clinical indications for therapeutical or cosmetic reconstructive muscle surgery. There are three types of muscle tissue: smooth muscles in the stomach and intestines, cardiac muscle in the heart, and skeletal muscle throughout the body. Skeletal muscle, which we will focus on in this blog, majorly consists of myocytes, the multinucleated contractile muscle fibres.
Methodology of SMTE
While Skeletal muscle tissue engineering (SMTE) has been introduced to the world of biomedical engineering for more than two decades, it remains a significant challenge, with numerous techniques being developed constantly to accomplish this.
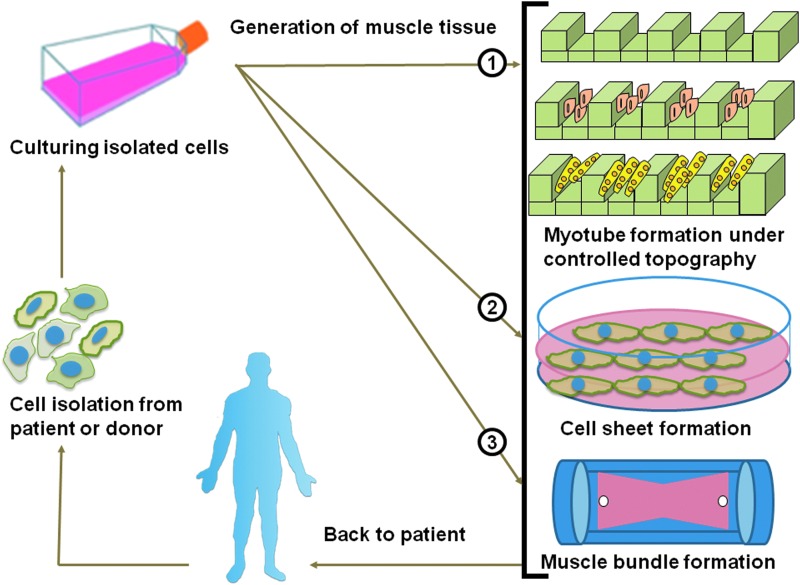
The muscle cells are first isolated from the patient or a donor, followed by culturing, where the cells effectively differentiate and develop in the nutrition-rich medium. The muscle tissue is then generated in three methods, which form different specialized muscle cells: myotube formation under controlled topography, cell sheet formation with a hydrogel matrix, and muscle bundle formation. The well-developed and functional tissue is then transplanted back to the patient.
Limitations
This is the traditional way of engineering skeletal muscle tissue, but it still faces some limitations. Though cells could be isolated from the patient, the tissues generated from a donor could trigger immune responses in the recipient’s body, causing rejection and the necessity for immunosuppressive drugs. These medications come with their own risk, like increased susceptibility to infections and organ damage. Moreover, traditional tissue engineering often focuses on preparing damaged muscle tissue rather than promoting regeneration. Thus, the regenerative potential of the SMTE tissue may be insufficient to restore full muscle function, especially in cases of extensive injury or degeneration quantitatively and qualitatively due to ageing. On the other hand, ensuring the long-term functionality of transplanted muscle tissue is also a significant challenge. Without adequate vascularization and support structures, it may encounter degradation or necrosis over time, leading to repeated interventions.
3D Print Muscle Tissue
I came across a piece of news published in 2023 written by Michael M., mentioning the researchers at the Terasaki Institute for Biomedical Innovation have developed a fascinating new technology of 3D printing skeletal muscle tissues with bioink invented by the researchers, which could mimic the natural muscle formation.
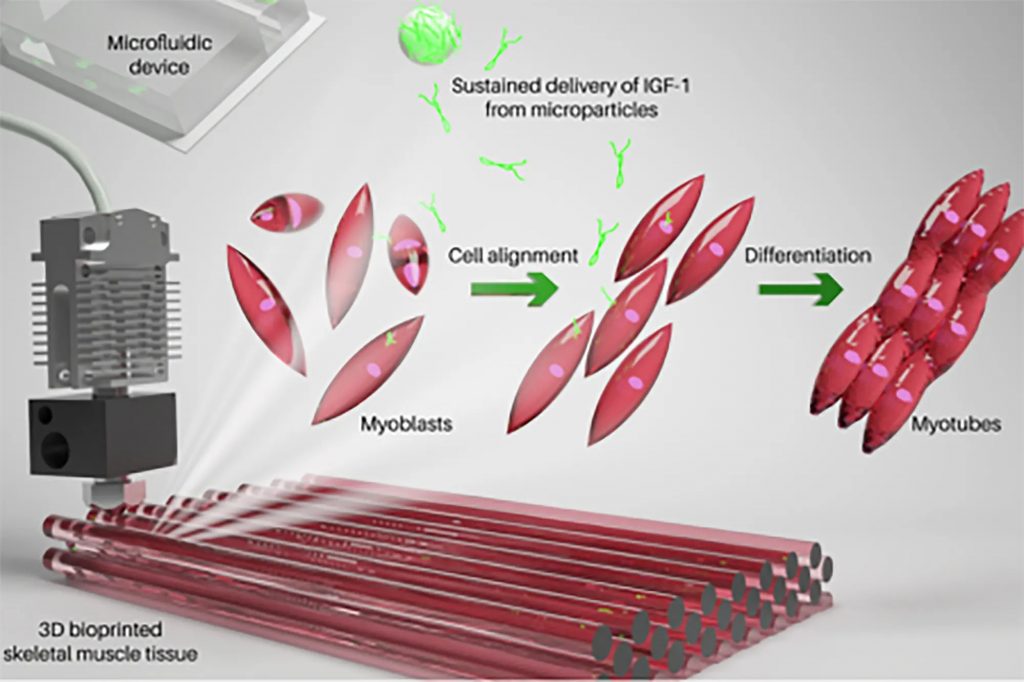
The science of muscle 3D printing
The three parts of the bioink are polylactic acid (PLGA) microparticles, myoblast cells, and a hydrogel based on gelatin. The PLGA microparticles sustainably release growth factor-1 (IGF-1), an insulin-like hormone that’s necessary for healthy bone and tissue growth. The production process of muscle tissue is more complex than it looks. Myoblast cells were deemed viable three days after printing and successfully developed into complete synthetic muscle tissue over the next ten days, eventually contracting on their own, just like normal muscle tissues! In order to determine if the cultured tissue was viable in living organisms, the tissue was implanted into mice. The researchers were then able to confirm that the cultured tissue was successfully accepted by the body and combined with the existing muscle tissues.
Summary
The ability to 3D print muscle tissue represents a significant advance in medicine. Using this technology, muscle mass replacement could be revolutionized by mimicking the natural process of muscle development. Prior to the possibility of treating humans, further clinical studies and testing will be necessary to confirm the safety of this process before the possibility of treating humans. What’s for sure, however, is that 3D printing muscle tissue has a highly promising future in medicine. I, as a biomedical science student, am also looking forward to the further development of skeletal muscle engineering.
References:
- Ostrovidov, S., PhD et al. (2014). Skeletal Muscle Tissue Engineering: Methods to Form Skeletal Myotubes and Their Applications. Tissue Engineering Part B Review, 20(5), pp. 403-436. doi: 10.1089/ten.teb.2013.0534
Very well written, with an excellent format and images. You’ve included interesting statistics and related it to personal ideas which…
This is a very well written blog, the format is as if you are talking directly to me. The ideas…
Love the Batman GIF :)
This is an excellent, well written blog. The narrative is engaging and easy to follow. It could be improved by…
This is a well-communicated blog. The it is written well with good use of multimedia. It could be improved with…