I have had an interest in stem cells since beginning my degree. Following a lecture on stem cells I attended, I found myself more curious about their applications and how they are attained. I was told umbilical cords are a good source of embryonic stem cells which are pluripotent (the most useful!) and my mum in fact told me she left my umbilical cord and placenta with the nurses to be used to harvest stem cells for those who need it. These cells are typically used in cancer, immune deficiency and genetic disease treatments. I found this super fascinating and I was proud that I, somewhat, contributed to that.
Stem Cells in Therapies
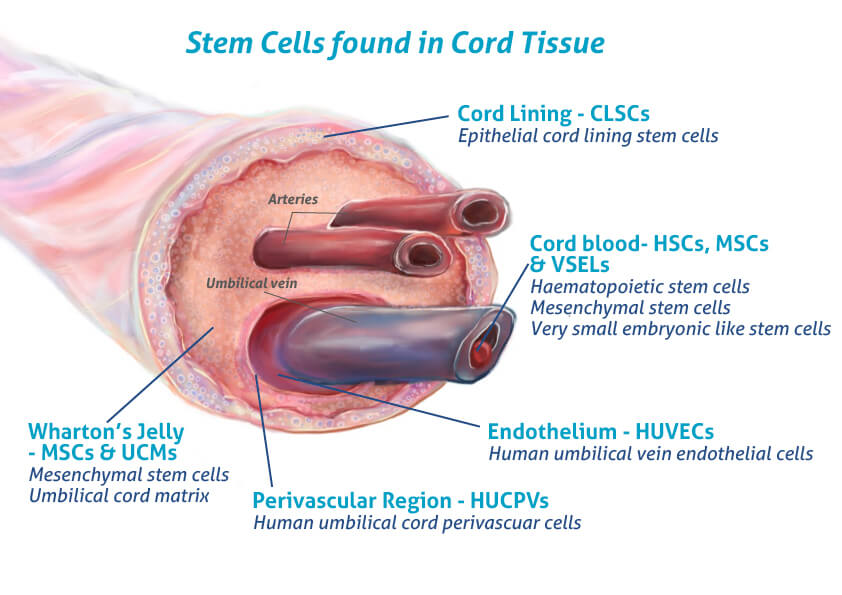
Stem cells used in disease treatment are already naturally lineage restricted, such as the transplantation of haematopoietic stem cells for blood cancers as discussed in our lecture. At the same time, some therapies may benefit from more undifferentiated stem cells, like human embryonic stem cells (hESCs) or potentially more ethically attained induced pluripotent stem cells (iPSCs). The limitations of using these pluripotent cells is that they result in the creation of a heterogeneous mixture including undesired cells, which as discussed in an article I saw, are difficult to expand and maintain in vitro, making in vivo applications more difficult. Further advantages and disadvantages of these cells are shown in the following image:

Scientists have weighed up these pros and cons and are now creating a different kind of stem cell that is much more specific for those cells that don’t have endogenous multipotent stem cells already.
Parkinson’s and Stem Cells
To investigate this further, I looked specifically at the use of lineage restricted stem cells in the treatment of Parkinson’s Disease (PD) which I have a personal interest in. For context, PD is a neurodegenerative disease characterised by progressively worse tremors and slow movements. It is unclear its direct cause, but stem cells are a trending mode of treatment. Scientists use pluripotent stem cells and treat them to become dopamine-producing neurones. Following legal guidelines from the Human Fertilisation and Embryology Act 1990, researchers have used embryonic cells from IVF programmes before 14 days of embryonic age. They also used iPSC since their discovery. But, these do not cure Parkinson’s. So what can be done to try to cure this disease?
An article I saw on researching this issue discusses their success with the use of lineage-restricted undifferentiated stem cells, from pluripotent cells. They found that 69% of these cells differentiated into the desired cell type (dopamine releasing neurones), a significant number compared to only 25% of hESC. This is a great result! They have kickstarted the research into lineage-restricted stem cells for PD (and I’m sure it has inspired people to try the method for other diseases )and emphasises how stem cell engineering is becoming a vital tool and could lead to potential cures for various diseases. I learnt there is more to stem cells than just pluripotency and they are more intricate than I had thought.
My final thoughts

I believe stem cell engineering and the potential around the creation of the ‘ideal stem cell’ is something that more people need to be educated on. There are still unresolved ethical questions but only through more education and understanding will we find the best ways to manage all expectations. It is exciting to think about the future applications of stem cells, what discoveries will be made and the overall benefits to all from this work.
Very well written, with an excellent format and images. You’ve included interesting statistics and related it to personal ideas which…
This is a very well written blog, the format is as if you are talking directly to me. The ideas…
Love the Batman GIF :)
This is an excellent, well written blog. The narrative is engaging and easy to follow. It could be improved by…
This is a well-communicated blog. The it is written well with good use of multimedia. It could be improved with…