Despite 100,000 people experiencing a stroke each year in the UK alone and statistically one in four people over the age of 25 having a stroke in their lifetime, there is currently no clinically effective treatment available to reverse the brain damage caused by strokes.
Last summer my mother had a stroke. Not long ago, this sentence filled me with both sadness and fear of what this actually meant, something not only I was feeling. The road to recovery for stroke patients can be steep and unknown due to the short time treatment can be given within, and the limited options currently available. This led my opinion of stroke research to be pretty negative and views of progress to be at a standstill.
A stroke is a life-threatening condition that occurs when the blood supply to parts of the brain is cut off, this lack of blood means no oxygen and nutrients can reach the brain leading to cell death. Strokes are medical emergencies, however, if caught early and treated patients are more likely to make a full recovery with almost no permanent disabilities.
The current treatments available in the UK depend on whether the stroke was ischemic; when blood supply to a region of the brain is blocked, or haemorrhagic; when there is excessive bleeding in parts of the brain. Other factors also include the time since the symptoms began and the presence of any other medical conditions.
Roughly 87% of all strokes are ischemic!
Treatments for an ischemic stroke include both medical procedures and medications. Mainly a medicine called a tissue plasminogen activator is used, which breaks up the blood clots that are restricting flow to the brain.
Whereas, for a haemorrhagic stroke blood pressure medicines are normally prescribed to lower the pressure on blood vessels alongside some form of procedure such as aneurysm clipping, coil embolisation, draining excess fluid and surgery to temporarily remove part of the skull.
Although there are multiple treatments available none are specifically tailored to each patient and can specifically target damaged areas regardless of the time taken to receive care. So, what if there was a way to precisely target damage no matter how long after the initial stroke?
A New Potential Delivery System…
In 2018, the University of Minnesota McAlpine research group projects 3D-printed a neuronal spinal cord scaffold.
Recent studies by Baker (2009) and Bible et al. (2009) aimed to develop technology for stem cell delivery to treat brain damage. They successfully attached neural stem cells to scaffold particles, optimised conditions for cell viability, determined the ideal particle size for effective delivery, and used MRI-guided implantation for precise targeting. Observations showed primitive tissue formation within seven days post-implantation, marking significant progress in developing stem cell scaffold matrices for stroke treatment. The study highlighted the potential of stem cell research to enhance transplantation viability using bio-scaffolding.
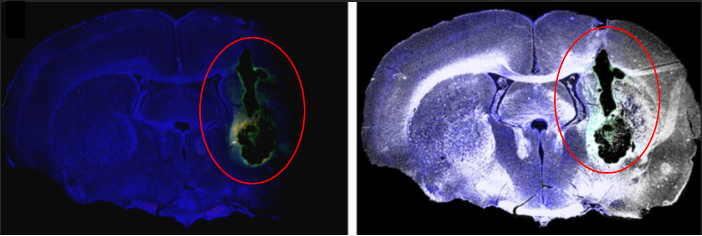
Researchers implanted neural stem cell-coated polymer particles into rat brains with simulated strokes using MRI-guided needles. Some cells formed a fibrous web within the graft, relying on blood vessel support for long-term survival. Post-mortem MRI images highlighted the grafted area, showing new tissue growth in green fluorescence.
Delving into stroke research has been an eye-opening journey for me, exploring the complex world of neurological damage and potential new treatments. It’s captivating to realise the immense challenges faced by individuals like my mother and other stroke survivors including the impacts on their lives and families, all adding to the urgent need for effective treatments. Investigating the latest developments in stem cell therapy, tissue engineering, and imaging techniques has left me both impressed and inspired for the future of stroke therapeutics.
Very well written, with an excellent format and images. You’ve included interesting statistics and related it to personal ideas which…
This is a very well written blog, the format is as if you are talking directly to me. The ideas…
Love the Batman GIF :)
This is an excellent, well written blog. The narrative is engaging and easy to follow. It could be improved by…
This is a well-communicated blog. The it is written well with good use of multimedia. It could be improved with…