As an aspiring Clinical Embryologist, I was particularly interested in our lecture on tissue engineering and promoting tissue regeneration. In the reproductive system, ovaries are generally the first to suffer decline in function over time. With a growing number of modern women seeking to beat age-related declines in fertility levels, there is much potential in using regenerative medicine strategies to restore ovarian function and overcome infertility. In 2016, success in a new experimental technique to increase fertility potential was published, using platelet-rich plasma (PRP), termed ovarian rejuvenation.
How does ovarian rejuvenation work?
PRP treatment has been used to treat osteoarthritis and joint degeneration. It involves separation of a patient’s blood sample via a centrifugation process to remove red and white blood cells to obtain a concentrated sample of plasma with a 5 to 10 fold higher concentration of platelets. Platelets are well known for their blood clotting abilities; however, they also facilitate tissue regeneration. Platelets contain granules which deliver various growth factors upon activation, including vascular endothelial growth factor (VEGF), platelet-derived growth factor (PDGF) and fibroblast growth factor (FGF). These promote vessel wall permeability and proliferation of endothelial cells and fibroblasts. Since 2016, interest has grown for applications of PRP in reproductive biology, where direct injection of PRP into the ovary has been reported to induce hormone stabilisation and resumption of menstrual cycles in infertile menopausal women.
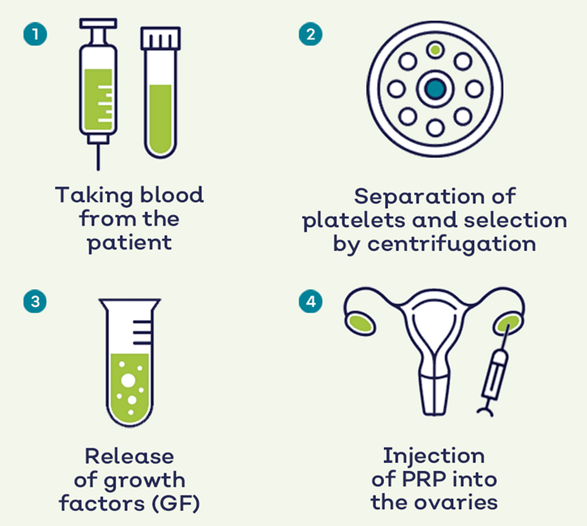
UK regulations
Whilst ovarian PRP treatment is actively in practice in countries such as Greece, USA and Turkey, it is not currently approved for in the UK by the Human Fertilisation and Embryology Authority (HFEA). Additional therapies and techniques such as ovarian rejuvenation are classified by the HFEA as fertility treatment ‘add-ons’. Although add-ons aim to improve fertility treatment outcomes, the evidence to support their efficacy and outcomes is usually missing, limited or lack reliability. With the UK fertility sector being a competitive market and where 60% of treatments are privately funded by patients, the HFEA is concerned that add-ons are offered for commercial interests rather than as best practice for patients. In December 2023, the Progress Educational Trust held a conference on ‘Updating Fertility, Embryo and Surrogacy Law’ where the HFEA proposed implicating fines as sanctions to fertility clinics for mis-selling of add-ons. Case studies of ovarian rejuvenation appear to present encouraging outcomes, however, there are often limitations in their experimental design such as by absence of sham injection groups or randomisation. The duration of effect after ovarian PRP is unknown, and potential short- and long-term risks are not yet understood. A large-scale, controlled randomised clinical trial is required to confirm efficacy of the therapy before ovarian rejuvenation may be introduced into UK clinics.
Ethical considerations
Ovarian rejuvenation has potential to improve chances of older and menopausal women conceiving their own biological child. However, some debate whether it is ethically acceptable to become a mother at an advanced age, given the likelihood of medical and health-related risks for both the mother and offspring.
I believe that such views are culturally skewed by ageism and ableism, leading to portrayal of older mothers as deliberately risk-taking when infertility and age of motherhood arises from life circumstances outside of a woman’s control. I hope that sufficient research may soon be conducted to prove efficacy of ovarian rejuvenation and its related regenerative therapies in fertility.
References
Pantos K. et al. Ovarian rejuvenation and folliculogenesis reactivation in peri-menopausal women after autologous platelet-rich plasma treatment: https://sa1s3.patientpop.com/assets/docs/111052.pdf
HFEA, The responsible use of treatment add-ons in fertility services: a consensus statement: https://www.hfea.gov.uk/media/kublgcp3/2023-10-19-treatment-add-ons-consensus-statement.pdf
Louisa Gheveart Associates, Fertility Law Reform In The UK: How Much Change Do We Want?: https://louisaghevaertassociates.co.uk/fertility-law-reform-in-the-uk-how-much-change-change-do-we-want/
Very well written, with an excellent format and images. You’ve included interesting statistics and related it to personal ideas which…
This is a very well written blog, the format is as if you are talking directly to me. The ideas…
Love the Batman GIF :)
This is an excellent, well written blog. The narrative is engaging and easy to follow. It could be improved by…
This is a well-communicated blog. The it is written well with good use of multimedia. It could be improved with…