Engineering Bronchial Tissue through the use of Scaffolds to Treat Asthma
Following the lecture on tissue engineering, I felt inspired by the emerging technologies and how these might lead to new therapies. I began to reflect on my own health condition of asthma, and became curious if this relatively-new innovation could be the answer to my future health. Having lived with this condition for almost 21 years, I have always wondered could we, one day, cure asthma entirely?
I began to research the future of tissue engineering in relation to lung conditions, and was particularly interested in Hafeji et al.,’s (2019) paper, Scaffolds for Tissue Engineering of the Bronchi, which has inspired this blog.
So what is Asthma?
Asthma is a common lung condition that causes occasional breathing difficulties. Symptoms include wheezing, a tight-feeling chest, breathlessness and coughing. During an asthma attack, airways become inflamed and the walls of the bronchi constrict, as you can see in the image below. This reduces airflow into the alveoli.
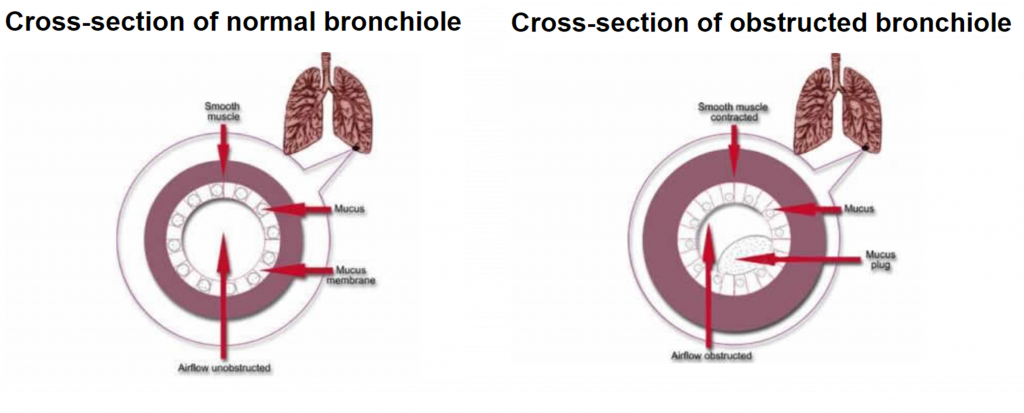
Triggers include infections, allergies, pollution and exercise, but attacks can also occur randomly, which is what I personally tend to find. Asthma affects more than 300 million people world-wide, so finding a cure is essential.
So what is Tissue Engineering?
Tissue engineering refers to the assembly of functional constructs that restore, maintain, or improve damaged tissues or whole organs. In this process, cells are selected, cultured and proliferated. For cells to proliferate, a scaffold is required. A large variety of cells are used for regenerative tissue engineering. These include:
- Allogenic cells = cells harvested from other individuals of the same species, including embryonic and mesenchymal stem cells.
- Autologous cells = cells harvested from the patient and reintroduced in a secondary site.
Tissue engineering in Asthma
Majority of bronchial tissue engineering studies have involved autologous and allogenic cells, including the use of human bronchial epithelial cells (HBECs) and human bronchial fibroblastic cells (HBFCs). The earliest study of this took place in 1999, where Zhang et al., seeded HBECs into a collagen-scaffold gel after HBFCs were incorporated, resulting in a tissue-engineered bronchial mucosa. This scientific success has lead to the continued study of bronchial disorders such as asthma.
Collagen – the best scaffold?
The role of scaffolds in bronchial tissue engineering is to provide an environment that resembles a native extracellular matrix, promoting proliferation and differentiation. When selecting the material for a bronchial scaffold, biocompatibility and strength must be considered.
Collagen is one of the most abundant structural proteins within tissues, offering high-strength, biocompatibility and even promoting cell proliferation. Even its fibre-structure is ideal -trapping growth factors and allowing HBECs to migrate and attach on the surface, hence allowing visualization under a microscope and study of bronchial disorders. Perfect, right?
Not quite… Collagen as a scaffold has limitations. Often extracted from animal tissues such as pigs or cows, collagen has poor immunogenic properties, posing a risk of carrying diseases.Therefore, collagen must be combined with immunosuppressants which increases cost. This use of animals also creates ethical and religious debate which I worry may limit the demographic that this innovation could target.
The Future
Despite these limitations, the use of bronchial tissue engineering should not be underestimated. I believe tissue-engineered bronchial mucosa acts as an effective three-dimensional model for the further study of this disorder and paves the way for the development of future effective therapeutic interventions, offering hope to millions of people world-wide, including myself.
If, like me, you feel inspired by this, check out Dr Kotton’s current and ongoing research into “rebuilding lungs“. I’m excited to see how this progresses, perhaps making use of tissue-engineered bronchial mucosa as a combined model. The future of asthma treatment looks bright.
Links/Sources:
- https://www.sciencedirect.com/science/article/pii/S0091674901404192
- https://utswmed.org/medblog/pediatric-asthma-tips-parents/
- https://www.nhs.uk/conditions/asthma/
- https://www.hse.gov.uk/asthma/lungs.htm#:~:text=Cross%2Dsection%20of%20normal%20bronchiole,actually%20block%20the%20smaller%20airways.
- https://cks.nice.org.uk/topics/asthma/background-information/prevalence/
- https://www.nibib.nih.gov/science-education/science-topics/tissue-engineering-and-regenerative-medicine#:~:text=The%20goal%20of%20tissue%20engineering,limited%20use%20in%20human%20patients.
- https://www.ncbi.nlm.nih.gov/pmc/articles/PMC2475566/
- https://www.sciencedirect.com/science/article/pii/B9780081025611000166
- https://www.biolinscientific.com/blog/what-is-biocompatibility
- https://www.medicalnewstoday.com/articles/vegan-collagen
- https://mokosh.com.au/blogs/blog/the-ethics-of-collagen-supplements#:~:text=A%20significant%20concern%20around%20using,a%20potentially%20less%20controversial%20source.
- https://www.anec.org/en/structure-of-collagen.htm
- https://pubmed.ncbi.nlm.nih.gov/16024520/#:~:text=The%20bronchial%20mucosa%20contributes%20to,membrane%20progressively%20unfold%20on%20inflation.
- https://www.bu.edu/articles/2023/stem-cell-therapy-lung-health-and-disease/
Very well written, with an excellent format and images. Youâve included interesting statistics and related it to personal ideas which…
This is a very well written blog, the format is as if you are talking directly to me. The ideas…
Love the Batman GIF :)
This is an excellent, well written blog. The narrative is engaging and easy to follow. It could be improved by…
This is a well-communicated blog. The it is written well with good use of multimedia. It could be improved with…